
Optimization of Accelerated Solvent Extraction of Polar and Non-polar Ginsenosides from Red Ginseng Using the Response Surface Methodology
Abstract
The objective of this study was to employ the response surface methodology (RSM) to model and enhance the accelerated solvent extraction (ASE) technique for the extraction of polar ginsenosides, non-polar ginsenosides, and total ginsenosides from red ginseng. To achieve this, a novel ASE-based method was developed and combined with RSM modeling and optimization. The RSM approach, utilizing a five-level, three-factor central composite design, was employed to determine the optimal combination of extraction parameters. In summary, the optimal extraction conditions for polar ginsenosides, non-polar ginsenosides, and total ginsenosides were as follows: an extraction solvent consisting of 88.64% ethanol, extraction temperatures of 82.30℃, 190.96℃, and 64.19℃, respectively, extraction times of 19.74, 28.77, and 28.77 min, respectively, and one extraction cycle. Under these optimized conditions, the experimental extraction yields for polar ginsenosides, non-polar ginsenosides, and total ginsenosides closely matched the predicted yields of 134.91, 68.86, and 149.35 mg/g, respectively, which were in excellent agreement with the model’s predictions. Therefore, to conclude, the present study provided a new and efficient method for the extraction of ginsenosides from red ginseng.
Keywords:
accelerated solvent extraction, red ginseng, polar ginsenosides, non-polar ginsenosides, response surface methodologyINTRODUCTION
Panax ginseng Meyer (Araliaceae), commonly referred to as Korean Ginseng, holds a prominent position in traditional medicine. The roots of Panax ginseng have been traditionally employed as a tonic to bolster the immune response and promote overall health and longevity (Im DS & Nah SY 2013). Ginsenosides, the active compounds in ginseng, are traditionally categorized into three primary groups based on their aglycone structure: protopanaxadiol (PPD) type, protopanaxatriol (PPT) type, and oleanane-type ginsenosides. Additionally, ginsenosides can be further divided into two groups based on their polarity: polar ginsenosides and less polar ginsenosides. In raw ginseng, the major ginsenosides belong to the polar group and include ginsenosides Rb1, Rb2, Rb3, Rd, Re, Rg1, Rf, and malonyl ginsenosides. Conversely, less polar ginsenosides such as ginsenosides Rg3, Rh2, compound K, Rh3, Rh4, Rk1, Rk2, Rk3, Rg5 are relatively scarce in raw ginseng products. Some studies have highlighted that the alteration of ginsenoside chemical structures induced by the steaming process significantly enhances the biological activities of ginseng roots. For example, during steaming, the concentration of polar ginsenosides decreases, while that of non-polar ginsenosides increases (Xie YY et al 2012). As a result of its relatively high levels of non-polar ginsenosides, red ginseng exhibits notably potent anticancer, antidiabetic, and anti-inflammatory properties (Yun TK et al 2001; Hong CE & Lyu SY 2011).
Accelerated solvent extraction (ASE) is considered an environmentally friendly technique for preparing plant material samples before chromatographic analysis (Azmir J et al 2013; Heng MY et al 2013). This approach, initially introduced by Dionex Corporation in 1995, is known by several names, including pressurized liquid extraction, pressurized solvent extraction, and enhanced solvent extraction. When water is used as the solvent, it can also be called pressurized hot water extraction, sub-critical water extraction, or superheated water extraction (Mustafa A & Turner C 2011). ASE is an automated, high-speed extraction method that uses minimal solvent while operating at elevated temperature and pressure. The increased temperature serves to enhance solubility and the transfer of solutes into the solvent, while elevated pressure prevents the solvent from reaching its boiling point. This combination enables rapid, safe, and efficient extraction of target analytes from plant materials into the extraction solvent (Mottaleb MA & Sarker SD 2012). Typically, an extraction process is completed in 15—25 min using only 15—45 mL of solvent. Consequently, ASE finds wide applications in various fields, including environmental, food, polymer, and pharmaceutical research (Hadidi M 2019).
Response Surface Methodology (RSM), initially introduced by Box GE & Wilson KB (1951), stands as a powerful statistical and mathematical technique for optimizing experimental parameters, making use of various experimental designs including the Box–Behnken design (BBD), central composite design (CCD), and Doehlert’s design (Zolgharnein J et al 2013). While it’s possible to approach the selection of optimal extraction parameters in a “one variable at a time” manner, this approach is notably time-consuming and often overlooks potential interactions among variables and parameters (Belwal T et al 2016). RSM serves as an effective statistical technique for optimizing complex processes, with CCD being a specific type of RSM that is particularly efficient, easy to set up and interpret for optimization experiments compared to other designs. CCD has found widespread application in optimizing numerous parameters (Viacava GE et al 2015; Belwal T et al 2016). The objective of this study is to utilize RSM to model and optimize ASE techniques for the extraction of polar ginsenosides, non-polar ginsenosides, and total ginsenosides from red ginseng. Several crucial factors, including the extraction solvent, extraction time, and extraction temperature, have been systematically analyzed using CCD.
MATERIALS AND METHODS
1. Materials
Six-year-old red ginseng purchased from Korea Ginseng Corp. (Daejeon, Korea). Ginsenoside standards, including Rb1, Rg1, Re, Rf, Rh1, Rc, Rb2, Rd, Rg6, F4, Rk3, Rh4, Rg3, Rk1, and Rg5, were procured from the Ambo Institute (Daejeon, Korea). Acetonitrile and water were provided by J.T. Baker (Phillipsburg, NJ, USA). All other chemicals utilized in this study were of analytical grade.
2. Accelerated Solvent Extraction
Pressurized liquid extraction was carried out using an ASE 350 System (Dionex, Sunnyvale, CA, USA). About 5 g of the red ginseng sample was evenly mixed with an equal weight of diatomaceous earth and placed into the extraction cell fitted with two cellulose filters. To prevent the powder from entering the extraction bottle, a frit and filter (Dionex Extraction Cell Filters, Dionex, Sunnyvale, CA, USA) were placed at the end of the cell. The ASE conditions were as follows: one static cycle, 60 solvent flush volumes, a 60-second nitrogen purge, and a pressure of 1,500 psi. Extraction variables for ginsenosides included extraction solvent, temperature, and static time. The resulting extract was subjected to a drying process through evaporation using a rotary evaporator at 50℃, after which it underwent freeze-drying.
3. Ginsenosides Analysis by HPLC
The ginsenosides were quantified following the procedure outlined by Dong H et al (2011) with minor adjustments. Analysis was carried out using an Agilent 1260 liquid chromatograph (Hewlett Packard, Wilmington, NC, USA) equipped with a quaternary gradient pump and multiple wavelength detector set to operate at 203 nm. Separation of samples occurred on a Zorbax Eclipse XDB-C18 column (4.6 mm × 150 mm, 5 μm; Agilent Technologies, Inc., Santa Clara, CA, USA) maintained at 35℃, and each sample was injected at a volume of 10 μL. The mobile phase consisted of a gradient mixture of water (A) and acetonitrile (B), with the gradient profile as follows: 20% B (0 min), 20% (0—10 min), 32% (10—40 min), 50% (40—55 min), 65% (55—70 min), 90% (70—82 min), and 80% (82—90 min). Data analysis was performed using Chemstation software (Hewlett Packard, Wilmington, NC, USA), and the mobile phase flow rate was set to 0.9 mL/min.
4. Experimental Design and Statistical Analytic
RSM was employed to optimize the extraction of ginsenosides from red ginseng, focusing on three critical factors: ethanol concentration (X1), extraction temperature (X2), and extraction time (X3). To enhance the experimental design, we applied an experimental designs (CCD) with six center points for these three factors. A five-level three-factor CCD was implemented using MINITAB Statistical Software (Release 21 for Windows, Minitab Inc., State College, PA, USA) to identify the optimal combination of extraction variables for maximizing ginsenoside yield from red ginseng. The CCD method encompasses factorial, center, and axial points, resulting in a design with five levels for each factor and three replicates, totaling 60 experimental runs [3(2k+2k+m)=3(8+6+6)=60]. Table 1 provides a comprehensive overview of the experimental plan, including both coded and uncoded levels of design factors. For each factor, we used codes of —1, 0, and +1 to represent the low, middle, and high levels, respectively. The lowest and highest levels were coded as —2 and +2, respectively. The mathematical relationship between the response variable Y and its corresponding factors is expressed through the following second-order polynomial equation:
Eq. 1 |
In this equation, Y represents the predicted outcome; β0, βj, βjj, and βij are the regression coefficients for the intercept, linearity, square, and interaction terms, respectively; Xi and Xj are independent coded variables. The estimation of response within each experimental design and the determination of the optimal conditions were conducted using MINITAB software. The adequacy of the polynomial model equation is assessed through the R2 coefficient. The F and P values were employed to assess the significance level (p<0.05, p<0.01, p<0.0001) of the regression coefficients. The data presented here represents the mean of three replicates. Furthermore, the experimental data underwent analysis of variance (ANOVA) to compare the mean values obtained from three replicate tests (i.e., p<0.05) using statistical analysis software (SPSS 20.0, IBM Inc., Armonk, NY, USA).
RESULTS AND DISCUSSION
1. Optimization of ASE by RSM
To assess the various influences of extraction variables on the combined content of polar ginsenosides, non-polar ginsenosides, and total ginsenosides, a BBD with five levels and three factors. The variables were characterized by the following ranges: for the extraction solvent (X1), ethanol concentrations of 21%, 35%, 55%, 75%, and 89% were considered; for extraction temperature (X2), values of 53, 81, 122, 163, or 191℃ were explored; and for extraction time (X3), a range from 5 to 29 min was investigated. These variables were categorized into five levels (—2, —1, 0, 1, and 2), resulting in a comprehensive experimental design comprising 60 data points. This included three replications at the central points, where all variables were designated as zero, as outlined in Table 1.
Table 2 presents the results of the analysis of polar ginsenosides, non-polar ginsenosides, and total ginsenoside content under various extraction conditions. Across 60 different conditions, which varied in ethanol concentration, extraction temperature, and extraction time, the polar ginsenoside content exhibited a range of 0.77 to 128.39 mg/g. The highest content was observed under the conditions of 89% ethanol concentration, an extraction temperature of 122℃, and an extraction time of 17 min (Run. 22), resulting in a content of 128.39±2.75 mg/g. In the case of non-polar ginsenosides, their content ranged from 6.39 to 57.58 mg/g, with the highest content recorded under conditions of 55% ethanol concentration, an extraction temperature of 191℃, and an extraction time of 17 min (Run. 9), resulting in a content of 57.58±1.24 mg/g. Furthermore, the total ginsenoside content spanned from 52.35 to 136.10 mg/g, with the peak content noted under conditions of 89% ethanol concentration, an extraction temperature of 122℃, and an extraction time of 17 min (Run. 46), resulting in a content of 136.10±2.92 mg/g. Notably, the sum of polar ginsenosides, non-polar ginsenosides, and total ginsenosides content displayed variations under different extraction conditions, as outlined in Table 1. To assess the relationship between variables and responses, second-order polynomial regression equations were formulated using RSM. The coefficients for linearity (X1, X2, and X3), quadratic (, , and ), and interaction (X1 X2, X2 X3, and X1X3) terms were computed, and their significance was determined using t-tests and associated p-values, as summarized in Table 3. Larger coefficients coupled with smaller p-values (p<0.05) indicated substantial influences on the respective responses. Furthermore, the correlations between the three independent variables and each response were assessed using multiple determinations (R2). The R2 values were calculated as 0.9792, 0.9806, and 0.99548 for the sum of polar ginsenosides, non- polar ginsenosides, and total ginsenosides content, respectively, underscoring the efficacy of this model. The model’s validity was substantiated via a lack-of-fit assessment (Table 3). The presence of non-significant p-values for the lack of fit (p<0.05) concerning the three responses affirmed that this model accurately represented the experimental data. Relationships between pairs of variables for the content of polar ginsenosides, non-polar ginsenosides, and total ginsenosides are visually portrayed through three-dimensional response surface plots, as governed by the regression equations (Figs. 1—3).

Experimental data on the sum of polar ginsenosides, non-polar ginsenosides, and total ginsenosides of red ginseng under different conditions based on central composite design for response surface methodology

Analysis of mean square deviation of regression equation for the sum of polar ginsenosides, non-polar ginsenosides, and total ginsenosides of red ginseng
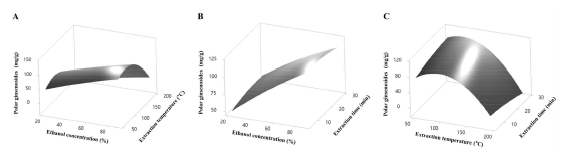
Response surface plot analysis of ethanol concentration, extraction temperature, and extraction time on the sum of polar ginsenosides. The fixed variables were set to coded value 0 as (A) 17 min, (B) 122℃, and (C) 55% ethanol.
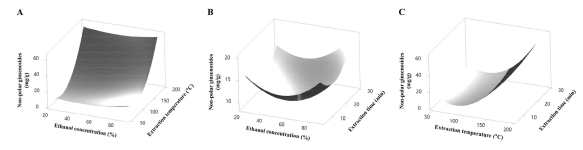
Response surface plot analysis of ethanol concentration, extraction temperature, and extraction time on the sum of non-polar ginsenosides. The fixed variables were set to coded value 0 as (A) 17 min, (B) 122℃, and (C) 55% ethanol.
2. Effect of Extraction Variables on the Sum of Polar Ginsenosides
Table 3 presents the design matrix and the respective outcomes from the RSM experiments. These experiments were carried out to evaluate the influences of three independent variables: ethanol concentration, extraction temperature, and extraction time. Utilizing multiple regression analysis on the experimental data, we can represent the anticipated response Y for the total sum of polar ginsenosides in coded values, as presented in the subsequent second-order polynomial equation:
where Y is the sum of polar ginsenosides (mg/g), and X1, X2, and X3 are the coded variables for ethanol concentration, extraction temperature, and extraction time, respectively.
Statistical evaluation of the model was carried out through analysis of variance (ANOVA). The ANOVA outcomes for the well-fitted quadratic polynomial model of the total sum of polar ginsenosides are outlined in Table 3. The quadratic regression model demonstrated a determination coefficient (R2) of 0.9792, along with a significant lack of fit at p<0.05. This indicates that the model’s calculations can aptly explain 97.92% of the observed outcomes. These results strongly suggest that the model employed to depict the response variable was highly significant (p<0.0001) and suitable for elucidating the connection between the response and independent variables (Hossain MB et al 2012). Additionally, the model’s significance was affirmed through an F-test, uncovering a remarkably high F-value (F=261.71). The adjusted determination coefficient, referred to as R2 adj, serves as a correlation measure to assess the quality of fit of the regression equation (Kim HK et al 2012). For this model, the R2 adj value was calculated as 0.9755, signifying that only 2.45% of the total variation remained unexplained by the model. The significance of each coefficient was determined using both the F-value and P-value (Table 3). Notably, two extraction parameters (X1, X2, p<0.0001 or X3, p>0.05) significantly influenced the sum of polar ginsenosides. Additionally, all quadratic parameters (, ) were significant at the levels of p<0.05 or p<0.0001, while was deemed insignificant (p>0.05). Moreover, the interaction quadratic parameters (X2 X3) were significant at p<0.01.
3. Effect of Extraction Variables on the Sum of Non-polar Ginsenosides
The equation for non-polar ginsenosides, expressed in coded values, was developed through a multiple regression analysis of the experimental data using a second-order polynomial regression model, as shown below:
where Y is the non-polar ginsenoside content (mg/g), and X1, X2 and X3 are the coded variables for ethanol concentration, extraction temperature, and extraction time, respectively.
Remarkably, the experimental model proved to be adequate (p<0.0001), and the lack of fit was notably significant (p<0.0001) (Table 3). The determination coefficient (R2) for non-polar ginsenosides reached 0.9806, signifying that the model could account for 98.06% of the variation. The model held high significance and aligned well with the experimental data. Furthermore, the positive linear effects of the two independent variables on all response variables were statistically significant (X1, p<0.01, X2, p<0.0001 or X3, p>0.05). Specifically, two extraction parameters (X1, p<0.01 or X2, p<0.0001) significantly influenced the sum of non-polar ginsenosides. Additionally, all quadratic parameters (, , ) demonstrated statistical significance at the levels of p<0.01, p<0.0001, p<0.01, respectively. However, the interaction quadratic parameters (X1 X2, X1 X3, X2 X3) were found to be statistically insignificant (p>0.05).
4. Effect of Extraction Variables on Total Ginsenosides
The equation for total ginsenoside, expressed in coded values, was developed through a multiple regression analysis of the experimental data using a second-order polynomial regression model, as shown below:
where Y is the total ginsenosides content and X1, X2 and X3 are the coded variables for ethanol concentration, extraction temperature, and extraction time, respectively.
Notably, the experimental model exhibited high adequacy (p<0.0001), and the lack of fit was determined to be non-significant (p>0.05) as shown in Table 3. The determination coefficient (R2) for total ginsenosides was 0.9548, indicating that the model accounted for 95.48% of the observed variation. This suggests that the model is highly significant and effectively explains the experimental data. Furthermore, the positive linear effects of the two independent variables on all response variables were highly significant (X1, p<0.0001, X2, p<0.0001 or X3, p>0.05). Two extraction parameters (X1, p<0.01 or X2, p<0.0001) had a significant impact on the total sum of ginsenosides. Additionally, only one quadratic parameter() was found to be highly significant at p<0.0001, while the interaction quadratic parameters (X1 X2, X2 X3) were also significant at p<0.01 and p<0.0001, respectively. However, the interaction X1 X3 was not significant (p>0.05).
5. Analysis of the Surface Plots
The interaction between two of the three variables and their impact on the recovery of polar ginsenosides, non-polar ginsenosides, and total ginsenosides content in a three-dimensional RSM plot (Figs. 1—3), while keeping the remaining three variables constant. Specifically, as the ethanol concentration increased from 21% to 89% at an extraction temperature of up to 86℃, there was a notable increase in the content of polar ginsenosides. However, as the extraction temperature continued to rise while maintaining a fixed extraction time, the content initially increased and then decreased. The peak content, at 134.80 mg/g, was reached when using an ethanol concentration of 89% and an extraction temperature of 86℃ (Fig. 1A). Furthermore, a similar linear effect was observed in relation to ethanol concentration, leading to an increase in the total polar ginsenosides content. The content of polar ginsenosides increased steadily until the ethanol concentration reached 89%. However, as the extraction time increased at a fixed extraction temperature, the content decreased. The optimal sum of polar ginsenosides reached its peak at 122.67 mg/g with an ethanol concentration of 89% and an extraction time of 8 min (Fig. 1B). In Fig. 1C, the interactive effect between extraction temperature and time, illustrating the negative quadratic effect of this interaction on polar ginsenosides content. The polar ginsenoside content showed a pattern of increase and decrease as both extraction temperature and time varied while maintaining a constant extraction concentration, with the peak content observed at 104.24 mg/g at an extraction temperature of 82℃ and an extraction time of 27 mins.
The influence of ethanol concentration and extraction temperature on non-polar ginsenoside content is visualized in the 3D response surface plot presented in Fig. 2A. When the extraction time remained constant, non-polar ginsenoside content exhibited slight fluctuations with increasing extraction temperature. Generally, higher extraction temperatures led to increased content, with the highest non-polar ginsenoside content observed at 62.72 mg/g, occurring at an ethanol concentration of 89% and an extraction temperature of 190℃. On the other hand, when maintaining a constant extraction temperature, the non-polar ginsenoside content experienced minor variations with changing extraction concentration and extraction time. Specifically, at an ethanol concentration of 89% and an extraction time of 29 min, the non-polar ginsenoside content measured 20.17 mg/g (Fig. 2B). Fig. 2C presents a three-dimensional response surface plot illustrating the interaction between extraction temperature and extraction time. The non-polar ginsenoside content exhibited fluctuations in response to changes in both extraction temperature and extraction time, all while maintaining a constant extraction concentration. Notably, the peak non-polar ginsenoside content was reached at 63.22 mg/g, occurring at an extraction temperature of 55℃ and an extraction time of 29 min.
Fig. 3A presents 3D response surface plots illustrating the impact of ethanol concentration and extraction temperature on the total ginsenosides content, as generated by the model. The total ginsenosides content exhibited a steady increase as ethanol concentration ranged from 21% to 89%, while keeping the extraction temperature below 80℃. However, it demonstrated an initial increase followed by a decrease with increasing extraction temperature, reaching its peak at 143.61 mg/g with an ethanol concentration of 89% and an extraction temperature of 80℃. Furthermore, an increase in ethanol concentration yielded a similar increase in total ginsenoside content. At a constant extraction temperature, the total ginsenoside content remained relatively stable or exhibited a slight decrease with prolonged extraction time. However, when the extraction temperature exceeded around 122℃, there was a noticeable decline in the total ginsenoside content. Additionally, the content showed a modest decrease as the extraction time increased at a fixed extraction temperature. With an ethanol concentration of 89% and an extraction time of 5 min, the total ginsenoside content measured 139.54 mg/g (Fig. 2B). However, the total ginsenoside content increased and decreased with the increase in the extraction temperature, while it increased as increase extraction time at a fixed extraction concentration, with the total ginsenoside content peaking at 112.66 mg/g at an extraction temperature of 55°C and extraction time of 29 min (Fig. 3C). The total ginsenoside content exhibited two distinct patterns in response to varying extraction conditions. It either remained stable or showed a slight decrease when the extraction time increased at a constant temperature. However, as the extraction temperature exceeded approximately 122℃, there was a noticeable slight decline in the total ginsenoside content. On the other hand, at a fixed extraction temperature, the content also exhibited a slight decrease with increasing extraction time. Notably, at an ethanol concentration of 89% and an extraction time of 5 min, the total ginsenoside content reached 139.54 mg/g (Fig. 2B). Conversely, the behavior of the total ginsenoside content varied when adjusting the extraction temperature while maintaining a constant extraction time. It exhibited both increases and decreases in response to changes in extraction temperature, but it consistently increased as the extraction time was extended at a fixed extraction concentration. The highest total ginsenoside content, peaking at 112.66 mg/g, was observed at an extraction temperature of 55℃ and an extraction time of 29 min (Fig. 3C). Chen Y et al (2007) observed that as temperatures increase, molecular mobility accelerates, leading to greater extraction efficiency. Additionally, higher temperatures enhance the solvent’s dissolution capacity while reducing surface tension and solvent viscosity. These factors collectively improve the mass transfer rate and, consequently, the availability of bioactive chemicals for extraction. However, it's important to note that saponin, as demonstrated by earlier research (Shi J et al 2004), is sensitive to high temperatures, which can reduce its extraction efficiency. Furthermore, Tan M et al (2013) found that excessive extraction time is unnecessary because the solvent and sample reach full equilibrium after a certain duration, following Fick’s second diffusion law. As equilibrium is achieved, the extraction process naturally slows down.
6. Determination of Optimal Conditions and Validation of the Model
To validate the accuracy of the model for predicting optimal yields, experiments were conducted using the recommended extraction conditions. The results closely matched the predicted values, demonstrating the reliability of the optimization process in this study. The quadratic polynomial regression model produced extraction conditions achieving a desirability score of 1.000. This desirability score, ranging from 0 to 1, indicates the performance of the variable, with 0 signifying an undesirable response and 1 indicating optimal functionality (Jeong IJ & Kim KJ 2009). The determined optimal extraction conditions for the sum of polar ginsenosides, non-polar ginsenosides, and total ginsenosides content were as follows: 88.64% ethanol in the extraction solvent, extraction temperatures of 82.30℃, 190.96℃, and 64.19℃, extraction times of 19.74, 28.77, and 28.77 min, along with a single extraction cycle. Under these optimal conditions, the sum of polar ginsenosides, non-polar ginsenosides, and total ginsenosides content reached 134.91, 68.86, and 149.35 mg/g, respectively (Table 4). To assess the model’s ability to predict actual values accurately, five verification experiments were conducted, resulting in values of 132.19, 67.70, and 144.18 mg/g, respectively, which closely aligned with the predicted values. The efficiency of ASE in saponin extraction was also evaluated and compared with other extraction methods. Güçlü-Üntündağ Ö et al (2007) reported that ASE resulted in a higher saponin yield from cow cockle seeds compared to ultrasonic-assisted extraction, using both pure and aqueous solvents such as ethanol and methanol. Similarly, Engelberth AS et al (2010) observed that the pressurized hot water system yielded a greater quantity of ginsenosides compared to the ultrasound-assisted method when extracting from Panax quinquefolium. Additionally, Wan JB et al (2006) noted that pressurized liquid extraction demonstrated distinct advantages in yielding a total amount of saponins, with a content of 7.36%, over other green extraction methods, including ultrasound (5.77%), conventional extractions using Soxhlet (6.99%), and maceration (6.00%), in the extraction of saponins from Panax notoginseng.
CONCLUSION
In this study, RSM was used to model and optimize the ASE technique to extract the sum of polar ginsenosides, non-polar ginsenosides, and total ginsenosides from red ginseng. This approach allowed for an effective assessment of the effect of three independent variables (ethanol concentration, extraction temperature, and extraction time) using contour and surface plots within RSM. In addition, a second-order polynomial model was utilized to optimize the extraction of ginsenosides from red ginseng through ASE technology. The optimized extraction conditions for the sum of polar ginsenosides, non-polar ginsenosides, and total ginsenosides were as follows: 88.64% ethanol in the extraction solvent, extraction temperatures of 82.30℃, 190.96℃, and 64.19℃, extraction times of 19.74, 28.77, and 28.77 min, along with a single extraction cycle. Under the optimum conditions, the experimental extraction yields of the sum of polar ginsenosides, non-polar ginsenosides, and total ginsenosides content agreed closely with the predicted yields of 134.91, 68.86, and 149.35 mg/g, respectively. Therefore, this study presents a novel and effective approach for red ginseng extraction.
Acknowledgments
This work was supported by the Kyungnam University Foundation Grant, 2023.
References
-
Azmir J, Zaidul ISM, Rahman MM, Sharif KM, Mohamed A, Sahena F, Jahurulb MHA, Ghafoorc K, Norulainid NAN, Omarb AKM (2013) Techniques for extraction of bioactive compounds from plant materials: A review. J Food Eng 117(4): 426-436.
[https://doi.org/10.1016/j.jfoodeng.2013.01.014]
-
Belwal T, Dhyani P, Bhatt ID, Rawal RS, Pande V (2016) Optimization extraction conditions for improving phenolic content and antioxidant activity in Berberis asiatica fruits using response surface methodology RSM. Food Chem 207: 115-124.
[https://doi.org/10.1016/j.foodchem.2016.03.081]
-
Box GE, Wilson KB (1951) On the experimental attainment of optimum conditions. J R Stat Soc Ser B Methodol 13(1): 1-45.
[https://doi.org/10.1111/j.2517-6161.1951.tb00067.x]
-
Chen Y, Xie MY, Gong XF (2007) Microwave-assisted extraction used for the isolation of total triterpenoid saponins from Ganoderma atrum. J Food Eng 81(1): 162-170.
[https://doi.org/10.1016/j.jfoodeng.2006.10.018]
-
Dong H, Bai LP, Wong VK, Zhou H, Wang JR, Liu Y, Jiang ZH, Liu L (2011) The in vitro structure-related anti-cancer activity of ginsenosides and their derivatives. Molecules 16(12): 10619-10630.
[https://doi.org/10.3390/molecules161210619]
-
Engelberth AS, Clausen EC, Carrier DJ (2010) Comparing extraction methods to recover ginseng saponins from American ginseng (Panax quinquefolium), followed by purification using fast centrifugal partition chromatography with HPLC verification. Sep Purif Technol 72(1): 1-6.
[https://doi.org/10.1016/j.seppur.2009.12.002]
-
Güçlü-Üntündağ Ö, Balsevich J, Mazza G (2007) Pressurized low polarity water extraction of saponins from cow cockle seed. J Food Eng 80(2): 619-630.
[https://doi.org/10.1016/j.jfoodeng.2006.06.024]
- Hadidi M (2019) Optimization of extraction of protein from alfalfa leaf (Medicago sativa) for human consumption. Ph D Dissertation University of Lleida, Spain. p 40.
-
Heng MY, Tan SN, Yong JWH, Ong ES (2013) Emerging green technologies for the chemical standardization of botanicals and herbal preparations. TrAC Trends Anal Chem 50: 1-10.
[https://doi.org/10.1016/j.trac.2013.03.012]
-
Hong CE, Lyu SY (2011) Anti-inflammatory and anti-oxidative effects of Korean red ginseng extract in human keratinocytes. Immune Netw 11(1): 42-49.
[https://doi.org/10.4110/in.2011.11.1.42]
-
Hossain MB, Brunton NP, Patras A, Tiwari B, O'Donnell CP, Martin-Diana AB, Barry-Ryan C (2012) Optimization of ultrasound assisted extraction of antioxidant compounds from marjoram (Origanum majorana L.) using response surface methodology. Ultrason Sonochem 19(3): 582-590.
[https://doi.org/10.1016/j.ultsonch.2011.11.001]
-
Im DS, Nah SY (2013) Yin and yang of ginseng pharmacology: Ginsenosides vs gintonin. Acta Pharmacol Sin 34(11): 1367-1373.
[https://doi.org/10.1038/aps.2013.100]
-
Jeong IJ, Kim KJ (2009) An interactive desirability function method to multiresponse optimization. Eur J Oper Res 195(2): 412-426.
[https://doi.org/10.1016/j.ejor.2008.02.018]
-
Kim HK, Do JR, Lim TS, Akram K, Yoon SR, Kwon JH (2012) Optimisation of microwave-assisted extraction for functional properties of Vitis coignetiae extract by response surface methodology. J Sci Food Agric 92(8): 1780-1785.
[https://doi.org/10.1002/jsfa.5546]
-
Mottaleb MA, Sarker SD (2012) Accelerated solvent extraction for natural products isolation. Methods Mol Biol 864: 75-87.
[https://doi.org/10.1007/978-1-61779-624-1_4]
-
Mustafa A, Turner C (2011) Pressurized liquid extraction as a green approach in food and herbal plants extraction: A review. Analytica Chimica Acta 703(1): 8-18.
[https://doi.org/10.1016/j.aca.2011.07.018]
-
Shi J, Arunasalam K, Yeung D, Kakuda Y, Mittal G, Jiang Y (2004) Saponins from edible legumes: Chemistry, processing, and health benefits. J Med Food 7(1): 67-78.
[https://doi.org/10.1089/109662004322984734]
- Tan M, Tan C, Ho C (2013) Effects of extraction solvent system, time and temperature on total phenolic content of henna (Lawsonia inermis) stems. Int Food Res J 20(6): 3117-3123.
-
Viacava GE, Roura SI, Agüero MV (2015) Optimization of critical parameters during antioxidants extraction from butterhead lettuce to simultaneously enhance polyphenols and antioxidant activity. Chemometr Intell Lab 146: 47-54.
[https://doi.org/10.1016/j.chemolab.2015.05.002]
-
Wan JB, Lai CM, Li SP, Lee MY, Kong LY, Wang YT (2006) Simultaneous determination of nine saponins from Panax notoginseng using HPLC and pressurized liquid extraction. J Pharm Biomed Anal 41(1): 274-279.
[https://doi.org/10.1016/j.jpba.2005.10.023]
-
Xie YY, Luo D, Cheng YJ, Ma JF, Wang YM, Liang QL, Luo GA (2012) Steaming-induced chemical transformations and holistic quality assessment of red ginseng derived from Panax ginseng by means of HPLC-ESI-MS/MS(n)-based multicomponent quantification fingerprint. J Agric Food Chem 60(33): 8213-8224.
[https://doi.org/10.1021/jf301116x]
-
Yun TK, Lee YS, Lee YH (2001) Anticarcinogenic effect of Panax ginseng C.A. Meyer and identification of active compounds. J Korean Med Sci 16(Suppl): S6-S18.
[https://doi.org/10.3346/jkms.2001.16.S.S6]
-
Zolgharnein J, Shahmoradi A, Ghasemi JB (2013) Comparative study of Box-Behnken, central composite, and Doehlert matrix for multivariate optimization of Pb (II) adsorption onto Robinia tree leaves. J Chemometr 27(1-2): 12-20.
[https://doi.org/10.1002/cem.2487]